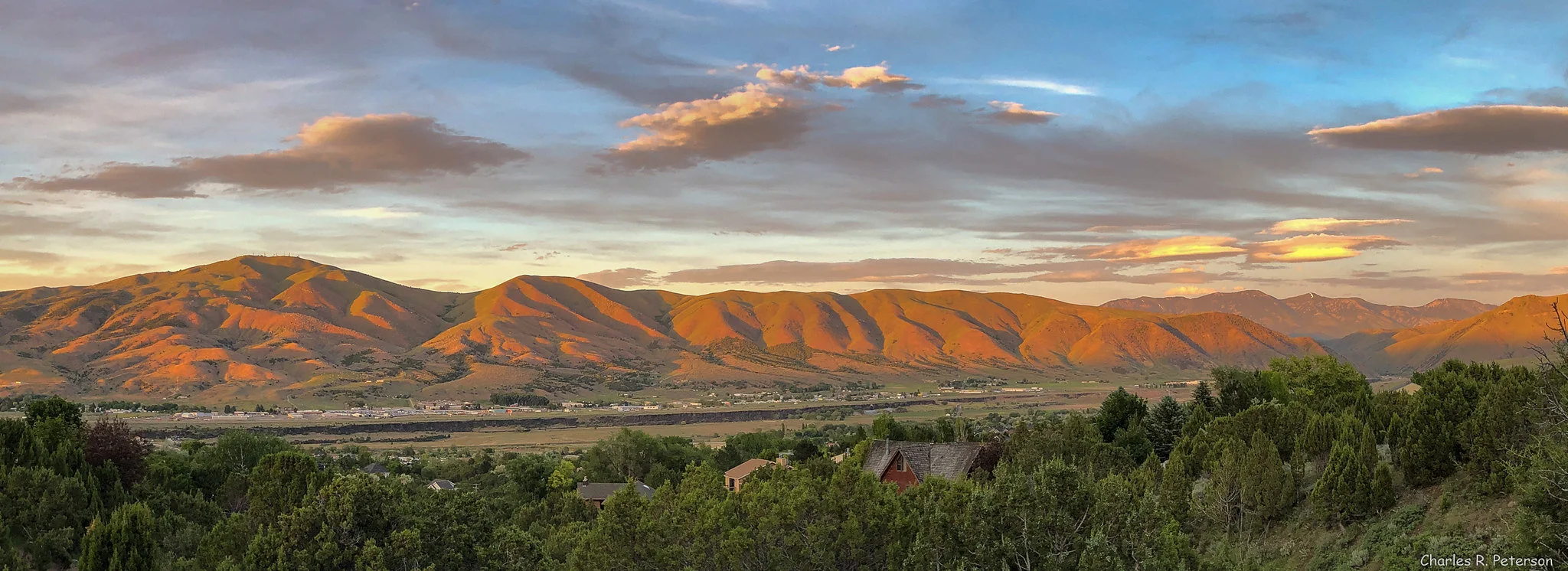
Current Initiatives
Confocal image of P. falciparum mitochondrion.
Image credit for header: Charles Peterson
P. falciparum mitochondrial gene regulation
In the human malaria parasite, Plasmodium falciparum, mitochondrial function is critically essential in both the human and mosquito life stages. Despite being a validated drug target for decades, most mitochondrial proteins and pathways remain uncharacterized. The lack of knowledge regarding basic functions and their regulation creates an enormous gap in our ability to exploit mitochondrial biology and biochemistry for targeted drug development. New approaches are necessary to identify highly conserved targets with a limited capacity to evolve drug resistance.
It is challenging to Investigate the malaria parasite mitochondrial components. There is only a single mitochondrion per parasite for most of the life cycle. The multicopy genomes are linear or circular, encode three essential electron transport chain (ETC) genes, but no tRNAs for translation. The proteome is uncharacterized and the parasite ETC complex subunits were only identified in 2020. P. falciparum mitochondria lack cristae to insert ETC enzymes, and it is unknown how gene expression is regulated. These are evidence that the parasite mitochondrion has evolved noncanonical means of gene expression and regulation compared to other eukaryotes. Ongoing lab efforts to identify and characterize these pathways are described below.
RECOMBINATION MECHANISM OF THE MITOCHONDRIAL DNA (mtDNA)
Resistance to ETC inhibitors is thought to be due to mtDNA mutation or recombination, however parasite mtDNA exists in linear and circular forms complicating the mechanism. Preliminary data shows exposure to atovaquone, a known ETC inhibitor, results in loss of circular mtDNA suggesting it recombined into the linear forms. The hypothesis is circular mtDNA encodes alternate or cryptic forms of the inhibitor target that can be rapidly recombined and expressed for parasite survival. The overall goals of this multi-pronged initiative are to 1) identify the cryptic forms of the mitochondrial genome and 2) elucidate the contributions of these to the evolution of drug resistance.
mtDNA TRANSCRIPTION MACHINERY AND REGULATION
The presence of multiple forms of mtDNA, known to recombine extensively, leads to the question of how recombination happens and how is recombination regulated? mtDNA encodes only three genes, which are part of the ETC, essential, and not duplicated on the nuclear chromosomes. We will use single-organelle proteomics to identify the uncharacterized mitoproteome including the mitochondria-specific DNA repair pathway. Identification of the transcriptional machinery will be validated using iterative rounds of targeted proximity labeling. This multipart project will define the previously unknown and uncharacterized mitochondrial DNA recombination and repair pathways, as well as the transcriptional machinery and regulatory proteins.
80α and SaPI1 virions. 80α has larger capsids (heads) to contain the 3X larger volume of DNA compared to the smaller SaPI1 virions. Image via Terje Dokland and reported in this VCU news article.
S. aureus bacteriophage genetics
Multidrug-resistant (MDR) Staphylococcus aureus infections are a significant health threat, causing a wide range of life-threatening cardiac and pulmonary infections. Most sequenced S. aureus strains encode at least one integrated prophage in the chromosome. After penicillin deployed, bacteriophage treatment of bacterial infections was quickly abandoned by Western medicine. The findings from this project will characterize novel pathways to trigger endogenous phage to lyse host cells, thus providing new ways to treat MDR S. aureus infections.
Using an 80α mutant unable to activate the late operon, I identified a new SaPI1-mediated mechanism that activates phage late gene transcription within the operon. The new late transcript initiates in the middle of the phage DNA packaging genes producing only half of the complex. The resulting virions cannot package host or phage DNA, and thus do not mobilize virulence or drug-resistance factors.
The goal of this project is to exploit a newly identified mechanism to activate phage late operon transcription without mobilizing the phage itself. Activating this switch results in bacterial lysis and prevents DNA packaging by disrupting the DNA packaging motor. The project weaponizes the highly prevalent, endogenous prophages against the bacteria in which they reside as a novel way to attack antibiotic resistant bacteria.